Deriving electron densities from linear polarization observations of the white-light corona during the 2020 December 14 total solar eclipse
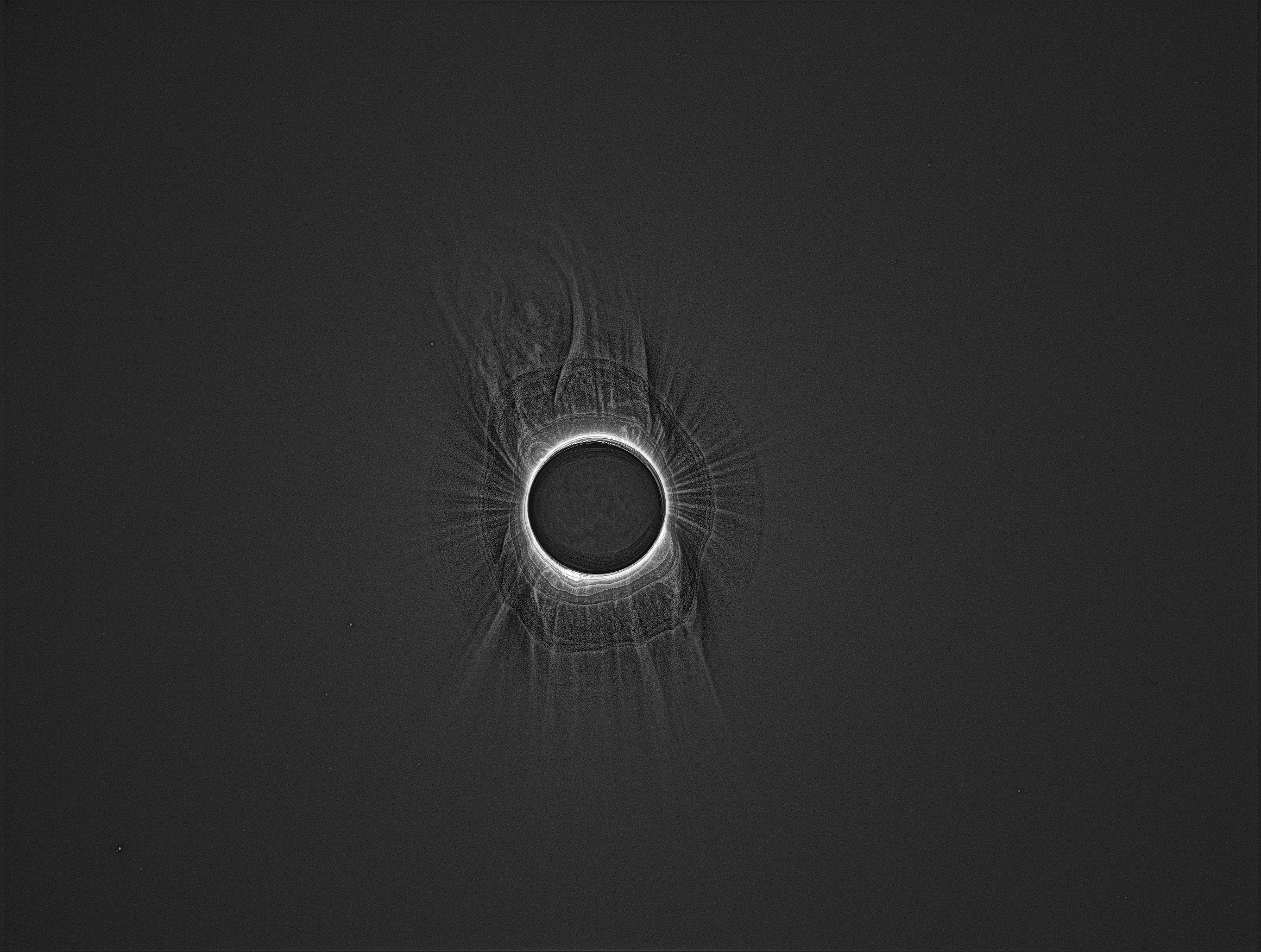
One of the important physical parameters to determine when studying the solar corona is the electron density. This can be determined by taking white-light polarisation images of the corona during the totality of a total solar eclipse. Various image processing techniques can then be used to bring out as much information in the images as possible, followed by an inversion technique, originally developed in the 1950s, which can be used to determine the electron density from the brightness of these polarised images. I am part of the team which sent an instrument - the Coronal Imaging Polariser (CIP) - to the total solar eclipse which occurred on December 14th, 2020, in South America. The instrument was named CIP since, in Welsh, to take a "cip" at something is to take a quick look - fitting since totality during a total solar eclipse typically only lasts a few minutes!